Quantizing the Nucleus: Maria Goeppert-Mayer and the Creation of Nuclear Shell Theory
- Dale DeBakcsy
- May 23, 2023
- 8 min read
How does radioactive decay know when to stop? When Uranium-238 breaks up, it goes through twenty-two intermediate isotopes before finally coming to rest at Lead-206, but why remain there? What's so special about Lead-206, or Calcium-48, or any of the other isotopes that nature seems to particularly favor? The answer lies in reimagining the nature of the nucleus, and creating a quantum model for it to match that of the electrons surrounding it. It's the realm of Magic Numbers and Waltzing Nucleons, ruled over by a woman who no sooner entered nuclear physics than she made its most important post-war theoretical discovery, Maria Goeppert-Mayer (1906-1972), the Madonna of the Onion.
Goeppert-Mayer's life is a tale of inertia overcome, both institutional and personal. For most of her life, she worked without salary or a title commensurate with her abilities, doing brilliant things when given the chance and then promptly being forgotten about for decades at a time. Her childhood was spent in a Germany that, while still maintaining a certain Prussian reluctance to let women into the sciences, had at least the example of Emmy Noether to light the way for those who would try. Maria was an only child in a family that boasted, on her father's side, six generations of university professors, and it was therefore expected, in spite of all social considerations, that she would become an academic herself. Her father actively encouraged her scientific curiosity, and the two had a tight relationship based on a spirit of intellectual adventure that sustained them as they traipsed over mountains and through forests seeking the riddles of nature.
She attended the University of Göttingen in the mid-Twenties, just in time for the Quantum Revolution to explode all around her, the momentum of that general excitement pushing her from a chosen career in mathematics to one in physics. She came under the patient and devoted tutelage of Max Born, and met an argumentative American named Joe Mayer who had come to Göttingen to learn the new physics at its source. He fell in love with her, as many did, but had the good fortune to be loved by her in return, beginning a partnership in science that would push them both into territory they might not otherwise have braved.
Maria was brilliant, but she also had a tendency to drag her heels when it came time to write up her work. Whether through an excess of rigor or modesty, at critical moments in her life she sat on important research, giving others the chance to snatch her discoveries from her. Her PhD work, hypothesizing the ability of an atom to absorb two photons at once, especially when exposed to high intensity light, could not be proven experimentally (laser experiments wouldn't confirm it for another three decades), but her argumentation was a model of precision, and it was Joe Mayer who kept at her to finish the writing and take her deserved degree.
Joe and Maria married, and moved to America, where Joe had a position at Johns Hopkins waiting for him. For Maria, however, the best that could be found was a small space to think and a pittance of a couple hundred dollars for doing it. She arrived in America in 1930, and didn't have an official, salaried, full-time university professorship to call her own until 1960, three years before winning the Nobel Prize, and ten years after having published her ground-breaking work on the Nuclear Shell Model.
In the meantime, she kept on top of quantum developments by talking with family friends Enrico Fermi and Edward Teller, and taking side work in physics as it offered itself, including writing a book on statistical mechanics with her husband that became a classic, and a stint doing top secret work for the atomic bomb project. It was a psychologically difficult time, her hatred of Hitler warring with her realization that the ultimate weapon she was helping to create might well be dropped on friends and family left behind. Even after doing important work on isotope separation and the effect of extreme temperatures on radiation, the best she could manage for years after the war was an unsalaried "volunteer associate professor" position at the University of Chicago, where Fermi had set up a group of nuclear experts and invited Joe Mayer to become a theoretical chemistry professor.

And yet, in spite of having been treated as essentially a Faculty Wife for the better part of two decades, she was at last within striking range of the work that would put her in the ranks of the quantum pantheon. She was interested in the peculiar stability of certain isotopes and, upon investigation, found that certain numbers kept popping up, what Eugene Wigner called "magic numbers." If a nucleus possessed a "magic" number of protons or neutrons, it tended not to decay, and was found in greater abundance than previous models could explain. Why did nature "like" these configurations so much? The few people who had studied this phenomenon before had found two such magic numbers, 50 and 82, scratched their head, and more or less let the matter lie, but Goeppert-Mayer wouldn't let the problem go. She went to the physicists working on the new cyclotron at Argonne and made use of the latest data which, subjected to her ruthless analysis, yielded a slew of new magic numbers: 2, 8, 20, 28, and 126 in addition to confirming the original 50 and 82. If a nucleus has a magic number of protons, it tends to be stable. If it has a magic number of both protons AND neutrons, it is "double magic" and is therefore very averse to decaying (Calcium is a particularly revered nucleus, as it has two double magic isotopes, Calcium-40 and Calcium-48).
Finding the new numbers using the latest experimental data was interesting, but finding a model that explained them was the real challenge. The common conception of the nucleus prior to this was a "droplet" that contained a grouping of neutrons and protons, but that model wouldn't explain the odd preference for certain numbers of nucleons (nuclear particles, be they protons or neutrons). That curious predilection looked distinctly quantum - in fact, a good deal like the model developed for electrons in the 1920s.
You might recall that certain configurations of electrons are "preferred" and tend to render an element less reactive once obtained, such as those possessed by the Noble Gases in the last column of the periodic table. But the case of electrons is at least somewhat physically intuitive - there seems a good deal of "room" for the electrons to move around outside of the nucleus, and therefore building up overlapping electron clouds and calculating the associated energy levels to find configurations of particularly low energy doesn't baffle the intuition too terribly much (though really, it ought).
But the nucleus? Where everything is packed together? Surely a series of onion-like shells in which the neutrons and protons orbit isn't applicable there? And indeed, the numbers didn't come out at first. Fermi and Goeppert-Mayer bounced ideas off of each other, and every time they thought they had made progress towards a model that explained nuclear behavior, an isotope would obstinately not fit their scheme. That is, until one day, when in passing, on his way out the door, Fermi said, "What about spin-orbit coupling?" It was a casual comment that he expected to go nowhere, but the force of its potential came all at once upon Goeppert-Mayer. She saw the mathematics working itself out in her head, and rushed to write it all out, finding that all of the magic numbers not only popped out of the theory, but were necessitated by it. The nucleons did have certain quantized shells into which their orbits fell.
Why, then, don't the protons and neutrons constantly collide? Because a collision requires there to be an empty quantum state that corresponds to the energy of the proposed collision. If all the quantum states are filled around the energy of a potential interaction, that interaction will not occur. There is, in quantum terms, nowhere to "shelve" it. Working out the energies of those nucleons allowed Goeppert-Mayer to show the combinations that had a uniquely high binding energy, and thus unusual stability and unusually high presence in the natural world - combinations that corresponded precisely with the magic numbers.
It was a mighty feat, and again she put off writing and publishing her work, at first out of a sense of fairness to some researchers who were working along similar lines, and then for reasons of self-doubt that, again, it was up to Joe to pull her out of. At the same time, it can be said that, without Maria, Joe would have probably remained an experimental chemist, and not made the successful transition to theoretical chemistry that made the whole second half of his career. Joe's assertiveness pushed Maria to write and publish, while Maria's knowledge and enthusiasm opened new intellectual vistas to Joe.
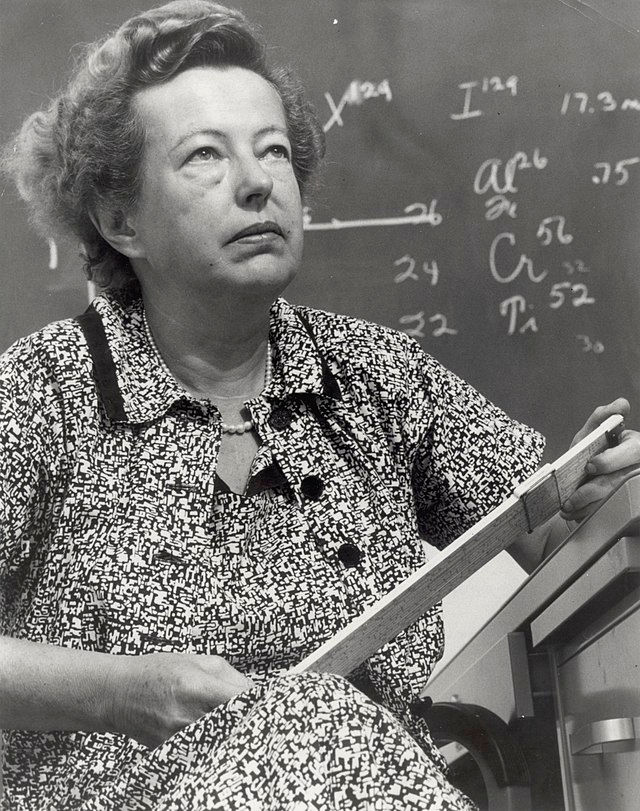
She finally published her full results in 1950, just as Hans Jensen was publishing his independently arrived-at shell theory. They would later collaborate on a book that wrote up their common results and served as a successor to the Statistical Mechanics text she had co-written with Joe. That book paved the way for the Nobel Prize thirteen years later, by which time she was finally a salaried professor working with Joe at the fledgling University of California, San Diego physics department. The rest of her life she tidied up the Shell theory, overseeing its application to the larger atoms, where it was discovered that the nuclei started taking on an oblong rather than spherical shape, but between suddenly declining health and old age, she ceased to produce the kind of insights that had made her a quantum hero.
Maria Goeppert-Mayer died in 1972, having spent just twelve of her sixty-six years in a position equal to her talents, and those the waning years. Had Johns Hopkins, Columbia, or the University of Chicago, institutions that readily hired Joe, found their way to doing more than the bare minimum in recognition of her talents, who is to say how much more she might have contributed to nuclear theory. On one hand, she was a theoretical physicist, for whom access to people is more important than access to a fully stocked lab, and Maria never had a problem in gathering brilliant people to bounce ideas off of, from Joe Mayer to Enrico Fermi to Max Born to Edward Teller. On the other, a life spent being seen as a Distinguished Wife, allowed attendance but not a vote at meetings with colleagues, denied decent pay for her work, undoubtedly took its toll on her confidence, with the result that she very nearly was scooped on the one project she did have the opportunity to work for a protracted stretch upon. She gave us the nucleus, and we gave her a volunteer associate professorship, and that is the full tragedy of it.
FURTHER READING:
For the level of her achievement, there is surprisingly little written, in German or English, about Maria Goeppert-Mayer. Daniela Wunsch has a short biography, Die letzte Physiknobelpreis fuer eine Frau? that is hard to track down, and pricey when you do. In the absence of that, I think the next best bet is a neat little book by Joan Dash called A Life of One's Own (1973). It features biographies of three women, each of about 110 pages, of which the last is that of Goeppert-Mayer, with a particular focus on the dynamics of her working relationship with Joe Mayer. The first biography is of Margaret Sanger, so bonus points there. The science presented isn't particularly technical, however, so if you're looking to go deeper into the Nuclear Shell Model, you'll probably want a text more devoted to quantum physics, which you'll have to approach from the electron side first, my favorite book for which being, as I've probably said before, David Griffiths's Introduction to Quantum Mechanics. It’s published by Pearson, who are basically evil and corruptive of the educational process on a global scale, but damn if it isn’t a good text.
This portrait was originally published as the 40th column of the Women In Science series, in 2015.
תגובות